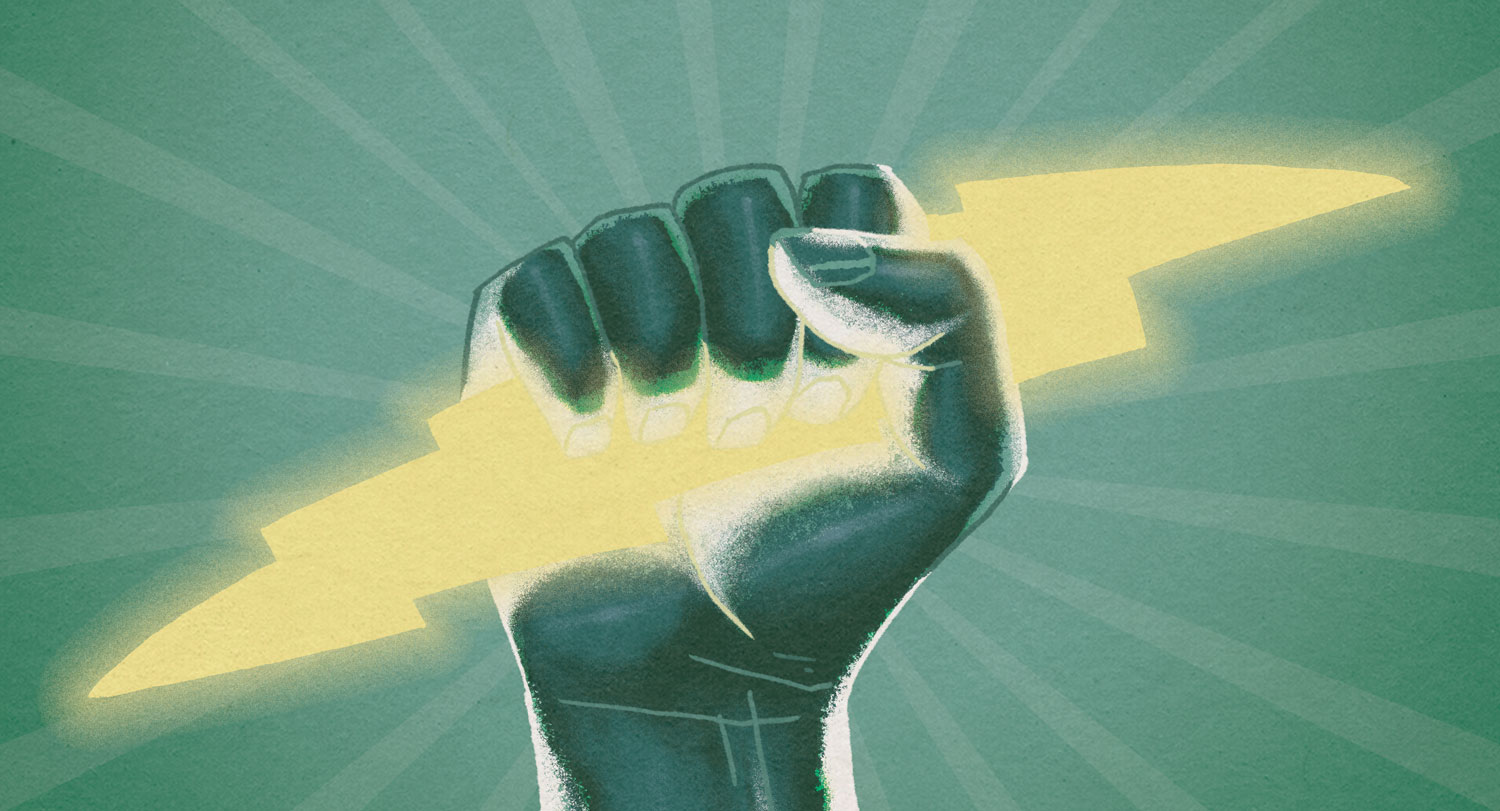
A leader in energy research, the University of South Carolina is part of the SC Nexus consortium, a regional technology and innovation hub for advanced energy research.
Widespread usage of solar panels and electric vehicles once seemed like the stuff of science fiction, but today it’s a looming reality. The U.S. Department of Energy projects that one in seven homes will have rooftop solar panels by 2030. By that point, electric car purchases could surpass more than 50 percent of total passenger car sales.
The world is poised to add as much renewable energy in the next five years as it did in the past 20, says the International Energy Agency.
But what will the transition toward cleaner energy sources mean for the job market? How can we harness hydrogen to make our transportation even greener? Could nuclear-powered rocket engines make interplanetary space travel more feasible?
Researchers at USC aren’t just seeking the answers to questions like these — they are actively influencing tomorrow’s energy landscape.
Finding a ‘just transition’ to clean energy policies and technologies
As the country transitions to more sustainable forms of energy production and consumption, what are the challenges and choices ahead for vulnerable populations and industrial workers?
Ann Eisenberg and Etienne Toussaint from the School of Law and Conor Harrison from the geography department are working to answer that, using a grant from the Alfred P. Sloan Foundation. The researchers, with colleagues at the University of Tennessee and the University of Pennsylvania, are studying what a “just transition” to cleaner energy technologies will mean to vulnerable groups in the South.
“This is a really ambitious undertaking,” Eisenberg says. “It’s a three-year, five-member team of interdisciplinary researchers at three institutions trying to bring the strengths of legal scholarship and geography to the table to tackle this huge problem that we’re facing right now of energy systems that are rife with injustice and inequities in a variety of forms.”
The research project, which started this summer, focuses specifically on South Carolina and Tennessee as case studies, as both states are experiencing energy transitions in regions with monopoly utilities that have long dominated the energy landscape. Both also are in areas where progress is being made toward transitioning to more sustainable forms of energy production and consumption.
“When I initially started working on energy, it was 2008-09 and the potential of renewable energy was there. But you could say things like ‘It’s so expensive,’ or ‘It’s sort of a fringe technology’ and you’d be right,” Harrison says. “But fast forward 13, 14 years and if you hear people say those things, they’re absolutely wrong. So there’s been really dramatic change in the ability to use renewable energy in a cost-effective way and have reliable technology. It’s not a fringe technology at all.”
That progress also can bring questions and challenges for vulnerable populations.
That includes, for example, how the job market will change when some forms of energy production, such as coal, are phased out and replaced with more sustainable forms. The researchers also will look at energy vulnerable households, meaning those that might struggle to pay energy costs or lack access to energy services.
“I think our effort is to really integrate in a meaningful and tangible way the experiences
of a variety
of different communities,” Toussaint says, “By stepping into the literal homes of
people who are experiencing energy vulnerability, by stepping into the factories of
workers, it will allow us to better understand the implications of energy transition
for a variety of different groups and the implications of law and policy reform. It
also will empower people at the grassroots level so that reform can reflect more of
a bottom up trajectory.”
Researchers will look at the laws and policies in place, how energy is produced and consumed and what efforts are already underway to promote sustainable forms of energy production and consumption in the communities. Their work is grounded in a belief that laws and policies focusing on energy systems must consider geography.
Once the project is complete, in addition to peer-reviewed articles reporting on findings and policy recommendations across research areas, the team also plans to produce community-oriented materials.
“We’ve been really intentional in thinking about how the things that we find in this project can be relevant to the communities where we’re doing the research,” Harrison says. “We want to work with these communities and work with our community partners to develop information and materials that are useful to them.”
That includes a handbook that could be distributed in the communities that could serve as a guide for influencing energy policy, offering concrete ideas on how people can get involved and who to contact for assistance on various issues.
“We’re trying to prioritize having this project be community-led and community-centered and ensuring that some of the fruits of the project go back to the communities,” Eisenberg says. “We hope for this to be a mechanism of empowerment for the partners and populations that we’re going to work with.”
Powered up
More than a decade before Energizer launched its battery-powered bunny in 1989, Ralph White launched his chemical engineering career with a special focus on battery design — and, just like the bunny, his research keeps going and going and going.
White, a chemical engineering professor in the College of Engineering and Computing, has been busy with nearly 50 years of battery design, improving several battery types including nickel-cadmium for early generation cordless tools, zinc-bromine for mass storage, nickel-hydrogen for satellites and lithium-ion and lithium-chloride for astronauts and other applications.
“My team doesn’t develop new materials, but we develop better battery designs through simulation,” says White, whose professional affiliations include NASA’s Electrical Power Technical Discipline Team, a safety oversight group. White also is a consultant for Comsol, a simulation software used throughout the battery industry for design improvement.
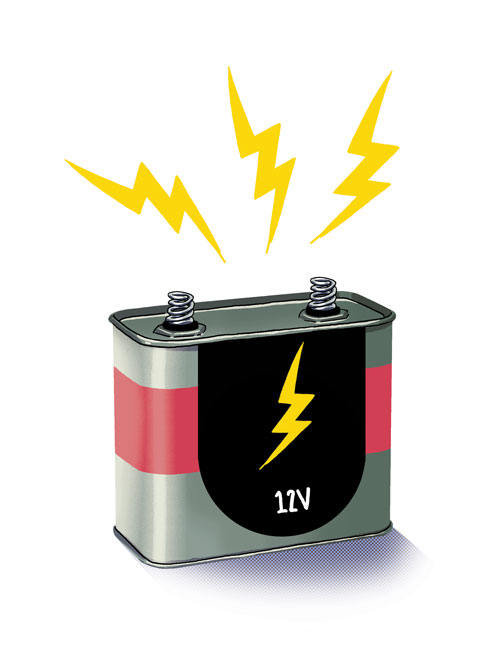
Li-ion batteries have emerged as the energy storage unit of choice for products ranging from cell phones and laptops to automobiles and airplanes, but safety issues remain a concern, he says. When li-ion battery cells are packed too closely together without design features to dissipate heat, a condition known as thermal runaway can lead to explosion and fire.
“Years ago, NASA had asked me about the safety of a battery pack for astronauts, and I told them I didn’t think it was safe because the 80 battery cells were too close together. ‘If one of them goes into thermal runaway,’ I said, ‘they’re all going to go, and it’s going to blow up and kill an astronaut,’” he recalls.
“They said, ‘White, you’re such a pessimist.’ Then the Dreamliner fire happened, a thermal run away event in the li-ion battery of an airliner, and suddenly NASA was asking me again about the astronaut battery pack. We spent a couple of years redesigning the pack to dissipate the heat more effectively and prevent explosion.”
1-1-1
It’s called the 1-1-1 Hydrogen Shot — a Department of Energy initiative to reduce the production cost of one kilo of hydrogen to $1 within one decade. Cheap, clean production of hydrogen is considered one of the linchpins in green energy.
Kevin Huang directs the university’s SmartState Center for Solid Oxide Fuel Cells, and that type of fuel cell technology has demonstrated promise in splitting water molecules to make hydrogen. If the process can be improved and made more efficient, it would be a quantum improvement over the current method of producing hydrogen by reforming or “cracking” methane (CH4), which produces nine kilos of carbon dioxide (CO2) for every kilo of hydrogen.
“Electrolyzers can split water molecules, which doesn’t release greenhouse gases, but so far the cost has been too high,” Huang says.
The Department of Energy has earmarked through the bipartisan infrastructure bill $1 billion for development and demonstration of new electrolyzers that can meet the 1-1-1 benchmark, and three types of devices have been considered.
Room temperature alkaline electrolyzers are about 60 percent efficient and produce hydrogen at a cost of $6 per kilo — three times more than the current $2 per kilo cost of reforming methane. Proton-exchange membrane electrolyzers, which use a proton-conducting membrane, operate at higher efficiency but their production cost of $5 per kilo of hydrogen and dependence on expensive noble metals such as platinum and iridium for catalysts makes them cost prohibitive.
“The third type of electrolyzer is solid oxide, and the advantage is that it operates at high temperature and the efficiency of splitting water molecules can reach 100 percent,” Huang says. “Some people think this technology can achieve the $1 per kilo of hydrogen benchmark.”
Huang has a $1 million grant from the Department of Energy’s fossil energy office to further refine the solid oxide fuel cell technology that he has been working on for years. A 2010 grant from DOE had allowed him to solve some of the earlier technology challenges.
The hat trick of hydrogen production would be to use energy created by renewable sources — solar, wind or hydro, for example — to power the electrolyzing process of splitting water. Then the hydrogen would be created without carbon emissions and the hydrogen itself could generate energy without carbon emissions.
While hydrogen-powered automobiles, buses and trains have long been in the public imagination, real-world applications for hydrogen have been more mundane. Most hydrogen currently is used to make fertilizer, refine petroleum and, increasingly, to make so-called “green” steel by using hydrogen to reduce iron oxide to iron, a process that reduces carbon emissions.
“To use hydrogen for transportation, the gas has to be pure because PEM fuel cells cannot tolerate carbon monoxide, which is a byproduct of reforming methane,” Huang says. “That’s another reason why forming hydrogen by splitting water would be beneficial: there’s no carbon involved so the hydrogen would stay pure.”
Next-gen nuclear
Along with 20 percent of U.S. electricity generation, nuclear power plants have, since the late 1970s, generated public opposition because of their exceptionally high construction costs, long construction time and concerns over safety and radioactive waste.
There is now an emphasis on so-called small modular reactors (SMR), which are projected to dramatically reduce costs and time to completion while allowing utilities to add capacity in more manageable increments. For almost two decades companies have been developing unique SMR designs, and this past summer the U.S. Nuclear Regulatory Commission certified the first one, designed by Oregon-based NuScale.
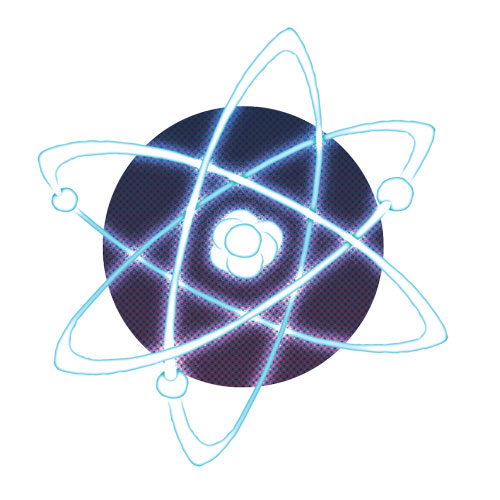
To address the need to handle future SMR radioactive waste concerns, the Department of Energy has funded a large research team, including scientists from the University of South Carolina, to devise a new way to process, compact, encapsulate and store the waste.
“Our focus is the waste that will be generated by small modular reactors, which will be a fraction of the size of the reactors that cost billions and were never finished here in South Carolina,” says Hanno zur Loye, a chemistry professor heading a USC team that’s part of a larger group funded by the federal energy department’s Advanced Research Projects Agency.
“The goal is to find a single method of nuclear waste processing that will be safe, stable and take up less volume than what we currently have. If this works, we will have a great process for taking care of the waste generated during electricity production, which is going to make utility companies more confident in buying these reactors and dealing with the waste.”
If successful, the three-year, $4 million project will result not only in new waste processing plans but also a prototype metal-clad cylinder that encapsulates the waste in ceramic form. Next-gen reactors could use different types of nuclear fuel, including metal oxides and molten salts, and zur Loye and his research team are tasked with developing a process for converting molten salt nuclear waste into a ceramic form.
“There are plenty of things we can substitute for radioactive materials in the proof-of-concept work. For the salts, the fact that they are not radioactive doesn’t matter — they’re still the same salt,” zur Loye says.
Ultimately, zur Loye and his research partners from across the country will look for an industrial partner to demonstrate that laboratory-scale processing of the nuclear waste can be carried out on a commercial scale.
“This is what I call a high-risk, high-return research project,” zur Loye says. “There are some real technical challenges ahead, and so there is a significant risk of failure — which ARPA is certainly aware of — but if our project succeeds, it could be a game changer.”
Rocket fuel
Engineering team studying advanced nuclear materials for interplanetary space travel
There is no question that space travel can be risky, especially a manned flight from Earth to Mars. But that risk could be reduced by cutting the time it takes for astronauts to make it to the Red Planet and back home.
That’s where mechanical engineering professor Travis Knight and his team are trying to help out. They are working on a way to develop mixed carbide fuels for nuclear thermal propulsion (NTP) for manned space flights, with the hope that it would increase efficiency, decrease costs and provide power for long periods of travel.
“There is much work to be done in terms of understanding the thermomechanical and thermochemical behavior under these very extreme conditions, because it involves high temperature, radiation damage and a corrosive environment. Those three things right there together, that’s a big challenge,” Knight says.
“We know that these perform better than some other candidate fuels. And that’s why we identified this as a leading technology for making this mission possible.”
The key, he says, is getting at the science of these materials — seeing how they behave in different environments and being able to predict that behavior with greater confidence.
“This is a new way of approaching space travel. These fuels are very high performing, but they also have different thermal and mechanical properties, and they operate in a different temperature and chemical environment. You wouldn’t want to get to Mars and your reactor be in pieces,” Knight says. “You want to make sure that we’re not sending our astronauts on a one-way trip.”
Along with South Carolina researchers, other entities involved in this project include the Los Alamos National Laboratory in New Mexico and NASA’s Marshall Space Flight Center in Huntsville, Alabama, which is funding the work.
This isn’t Knight’s first foray into the study of fuels for space exploration. During his doctoral studies, Knight researched space nuclear power, specifically mixed carbide fuels for nuclear thermal propulsion. And he has been studying nuclear fuels and materials in his lab on campus for the past 18 years.
Knight says working on fuel issues for space travel is exciting, in part because of its appeal to current and potential students. But this project also has far-reaching applications in advanced nuclear power closer to home.
“Yes, there is an excitement or cachet value for space applications, but the impact on Earth would be greater from more advanced nuclear applications, helping the environment and reducing pollution and all that,” he says. “It’s not just making electricity, it’s alternative fuels for transportation that have a very low carbon footprint.”
But space research — like all research in general — offers the opportunity to improve our understanding of the world and learn about ways to improve life on Earth.
“Sometimes there’s the question of what is the purpose of going to Mars or the Moon or any of these things. And it’s worth reiterating that there’s the basic understanding you get of being there, making observations, learning about the environment and what is applicable to life back on Earth,” he says. “There are resources to be had in these far-flung locations. And then there’s just the whole human ambition for exploring and for conquering new horizons. And I think that just continues to excite people.”